Story of discovery: how different medications for diabetes and obesity emerged from basic research on one pancreatic hormone
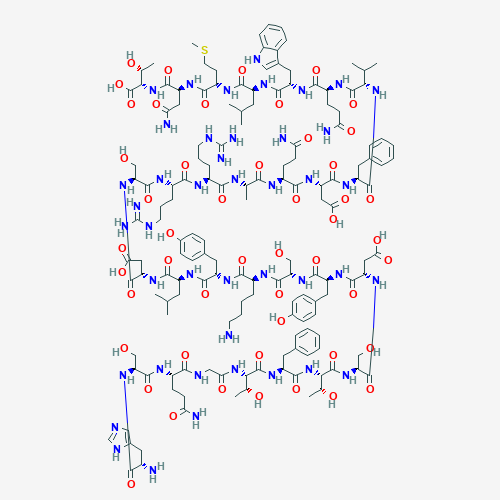
Basic research to understand and characterize the hormones controlling blood glucose (sugar) levels, including the discovery of the glucagon gene, ultimately led to two very important therapeutics with very different purposes. One of these therapies helps people with type 2 diabetes lower their blood glucose while reducing their risk of cardiovascular disease and also helps people with obesity lose weight; the other helps raise blood glucose levels in people with type 1 or type 2 diabetes if they develop hypoglycemia. Remarkable advances along the way came from studies in a variety of model organisms including not only typical lab animals like mice and rats, but also organisms like the deep-sea anglerfish and the desert-living Gila lizard.
Glucagon: Insulin’s Hormonal Opposite Is Also Its Essential Partner
In healthy individuals, the pancreatic “islets”—small, densely packed groups of several different types of cells—have a central role in maintaining optimal glucose levels in the body. One islet cell type, designated β (beta), responds to elevated blood glucose levels by releasing insulin, the hormone that induces cells to absorb sugar. When blood glucose levels are too low, another type of islet cells—α (alpha) cells—release a hormone called glucagon, which signals the liver to release glucose into the blood. These two critical hormones also regulate one another—rising insulin levels help inactivate lingering glucagon, and vice versa—to ensure agile transitions between periods of fasting and of caloric plenty. The net effect is to provide precise regulation of glucose levels within a narrow range that supports the optimal function and the long-term health of the body’s cells and organs. In diabetes, this perfectly choreographed regulatory dance is disturbed either by loss of the insulin-producing β cells (type 1 diabetes) or by inadequate amounts of insulin to compensate for a weakened response to the hormone by other cell types (type 2 diabetes).
The 1921 discovery that purified insulin could be used to treat diabetes meant that for the first time in history, children diagnosed with what we now call type 1 diabetes could live to adulthood. However, the dangers of over-treating diabetes soon became clear: depending on severity, hypoglycemia—low blood glucose levels—can cause symptoms ranging from clumsiness, irritability, or confusion, to lost consciousness, seizures, and even death. In contrast, high glucose levels may not cause acute symptoms until they are well above normal. Over time, however, high blood glucose levels have been shown to lead to the chronic complications of diabetes. The landmark NIDDK-supported Diabetes Control and Complications Trial (DCCT) changed this by showing that keeping blood glucose control close to normal is vital to slowing development and progression of long-term diabetes complications that can lead to disability and death. Researchers have long been vigorously searching for better ways to control blood glucose levels both safely and effectively.
Treating mild cases of low blood glucose levels is sometimes as simple as consuming a source of glucose, so schools, as well as individuals with diabetes who take insulin or certain other drugs that can cause excessive release of the hormone, often keep glucose tablets on hand for use in such situations. But one of the greatest concerns for people with type 1 diabetes, and those who love and care for them, is a drop in blood glucose levels that goes unnoticed because it occurs during sleep, and therefore can become extremely dangerous. Glucose tablets are only helpful, of course, when hypoglycemia is recognized and when it occurs in someone who is conscious and able to eat or drink, so more severe episodes of hypoglycemia can be deadly. In a hospital setting, intravenous delivery of glucose can be a life saver if hypoglycemia is noted. Similar interventions can sometimes be necessary for children with diabetes if they refuse to eat, or if another disease like the flu prevents someone with diabetes from keeping food down. In schools and homes, however, there have been few practical options. Moreover, the danger of hypoglycemia is a major source of anxiety not only for people with diabetes but also for their caregivers and is a serious obstacle to achieving optimal glucose levels for long-term health. Furthermore, repeated episodes of hypoglycemia can lead to a weaker compensatory glucagon release from α cells and also cause people to lose the ability to recognize hypoglycemic symptoms, increasing the risk for severe episodes. As a result, scientists have long recognized the potential of glucagon as an emergency medical treatment to combat dangerously low blood glucose levels.
Why did nearly 100 years pass after insulin came into widespread use to treat diabetes for its vital opposing partner in controlling glucose levels to start becoming more widely available and convenient to use? The factors that long frustrated therapeutic development of glucagon, and the innovations that are overcoming those problems, are part of a remarkable scientific story.
Fishing for Glucagon
In fact, researchers developed a process for isolating glucagon from an animal pancreas in 1923, just 2 years after the landmark purification of insulin. Indeed, a preparation of glucagon was approved by the U.S. Food and Drug Administration (FDA) in 1960 for the emergency treatment of hypoglycemia, but two characteristics limited the product’s therapeutic potential: the hormone rapidly forms insoluble, injection-clogging fibrils in water; and it quickly degrades if not kept frozen. Because it would be impractical to keep frozen, dissolved glucagon on hand at all times, the FDA approved the drug as a dried powder. Unfortunately, dissolving the hormone with sterile water and administering it in time and at the correct dose posed logistical challenges for non-medical professionals that limited its usefulness.
The first step to understanding and overcoming these problems was to find the glucagon-encoding gene; and the first glucagon gene to be identified came from what might seem a very unlikely source: the deep-sea-dwelling Atlantic anglerfish. These animals hide on the ocean floor, waiting for prey to come along, and glucagon is essential to maintain their glucose levels when that wait is a long one. But there was also a practical reason to study the hormone in anglerfish: their islets are encapsulated in easily identified and isolated structures, rather than hidden within the pancreas. Thus, anglerfish α cells proved to be a convenient source for the NIDDK-supported scientists who first identified the glucagon gene in 1982. Having the anglerfish version in hand facilitated the search for corresponding mammalian glucagon genes, so researchers soon identified rodent and human versions as well.
These advances made it possible to put the gene into laboratory bacteria to produce significant quantities of the human glucagon protein—called “recombinant” glucagon—so that producing the hormone became more efficient, and the preparations more easily purified. However, it was not until 1998, 5 years after publication of results from the DCCT establishing the importance of keeping blood glucose levels close to normal, that two different pharmaceutical companies developed recombinant glucagon as a commercial product and received approval from the FDA to make and market it for emergency treatment of severe hypoglycemia. Unfortunately, the recombinant hormone was just as likely as glucagon from other sources to form fibrils and degrade in water, so the barriers to its widespread use remained in place.
New Approaches for Glucagon Delivery—and New Needs
Two significant innovations facilitating use of glucagon were FDA approved for use in 2019. The first of these is a new method of delivering the hormone: by simply spraying the dried powder into the nose. This eliminates the need to dissolve it in water or inject it and therefore makes the prospect of giving the drug much less intimidating to untrained bystanders. The second, developed with NIDDK small-business grant support, takes the approach of dissolving the hormone in a non-toxic liquid called dimethylsulfoxide (DMSO), instead of water. Glucagon remains stable for months in room- temperature DMSO without forming fibrils. The DMSO-dissolved hormone is distributed in easy-to-use injector “pens” that automatically deliver an appropriate dose to raise glucose levels.
A need for smaller, non-emergency doses of glucagon is now arising as a result of another major advance in medical care for type 1 diabetes: development of “artificial pancreas” devices. In their simplest form, these devices continuously measure a person’s blood glucose levels and automatically administer an appropriate amount of insulin, if needed, to bring glucose levels down to healthful levels. This approach reduces the chances of developing hypoglycemia due to an accidental overdose of insulin. However, if glucose levels fall too low for any other reason—as a result of fasting, exercise, or illness, for example—the inability of such an insulin-only device to react by providing glucagon is a major limitation compared to a healthy pancreas. A “bi-hormonal” artificial pancreas—one that can respond like a functioning biological pancreas by lowering glucose with insulin or supplying a low dose of glucagon to raise glucose levels, if necessary—might therefore be a great boon to patients, adjusting blood glucose to optimum levels before they get into a range that would cause the wearer to experience symptoms or suffer any negative long-term health consequences. Today, several companies, often with vital NIDDK academic and small-business grant support, are developing and testing methods for delivering low-dose glucagon, using some of the same methods that are either approved or in development for use with emergency glucagon pens. In the future, such formulations may improve on already beneficial artificial pancreas products, leading to better, simpler care with lower risk of hypoglycemia for people who require long-term treatment with insulin.
An Unexpected Bonus Hormone Identified During The Hunt For The Glucagon Gene
The complementary effects of α and β cells and their signature hormones would seem to offer a simple, tidy explanation of the way the body controls blood glucose levels; but that explanation turns out to be incomplete in fascinating ways. In the 1960s, researchers were surprised to discover that the pancreas releases more insulin when a given amount of glucose is absorbed through the digestive system than if it is injected directly into the blood. The underlying reasons were a mystery, but the scientists speculated that the presence of glucose or other food in the gut might prompt the release of some different, unknown hormone that signals the pancreas to prepare for an increase in glucose levels by readying its supply of insulin. The scientists referred to such hypothetical insulin production- promoting hormones as “incretins,” but decades passed before work in other laboratories proved that this idea was correct.
In fact, the next clue to the incretin mystery was uncovered during the 1982 discovery of the anglerfish glucagon gene: an adjacent gene turned out to encode a protein that is notably similar to glucagon, yet clearly different from it. The researchers therefore called it a “glucagon-like peptide,” or GLP. Further work showed that, in contrast to fish, mammals do not have just two similar genes next to one another—they have three: the gene for glucagon, plus genes that came to be designated GLP-1 and GLP-2. Additional NIDDK- supported research later demonstrated that a truncated form of the product of one of these genes may be able to act as an incretin: pancreatic β cells release more insulin in response to elevated glucose if they are first stimulated with part of the GLP-1 protein. Thus, surprisingly, GLP-1 turns out to work somewhat in opposition to the very similar glucagon, helping to lower glucose levels by boosting the release of insulin. In another contrast with glucagon, experiments demonstrated that GLP-1 is produced not in the pancreas but by intestinal cells close to the stomach—and only when stimulated by the presence of food—helping establish that it is, in fact, an incretin. Experiments also demonstrated that GLP-1 has at least one other important effect on the body: it briefly slows stomach emptying, delaying digestion for a few minutes so that insulin will be ready for release before glucose levels have risen significantly.
Development of an Entirely New Class of Diabetes Medication
The effects of GLP-1 sound very much like properties one might like to have in a treatment for type 2 diabetes: by boosting insulin secretion in response to food, GLP-1 might bolster the natural insulin response of a person with the disease and thereby help keep glucose at healthier levels, while slowing digestion to allow this to happen. Unfortunately, scientists soon discovered a problem with the idea: GLP-1 only lasts a few minutes in the blood stream before it is degraded by other proteins. A therapeutically useful form of the hormone would therefore need to be much more stable in order to be a practical treatment for type 2 diabetes.
As it happens, scientists discovered a solution to this problem in what may seem like the unlikeliest of animals—a venomous lizard that lives in a habitat that could hardly be more different from that of the anglerfish, the U.S. Desert Southwest and Northwest Mexico. At about the same time that the glucagon-like peptides were being discovered by NIDDK grantees, NIDDK intramural scientists working with the lizard, called a “Gila monster,” discovered that mingling with the venom in the lizard’s saliva were proteins that appeared to “jump- start” its digestive system after the months of fasting that normally occur between its meals. Isolated from this protein mixture was a hormone they designated exendin-4 that was notably similar to glucagon, and even more akin to GLP-1. Scientists showed that exendin-4 and GLP-1 are both capable of stimulating gastric secretions in guinea pigs, though exendin-4 is the more potent of the two. They went on to show that both proteins work by stimulating the same cellular receptor, and that exendin-4 is a potent incretin. Importantly, a small but significant difference between the GLP-1 and exendin-4 structures protects the latter from digestion in the blood, where GLP-1 disappears within a few minutes, yet exendin-4 persists for about 12 hours.
Based upon these critical, NIDDK-supported basic science discoveries, pharmaceutical companies developed “exenatide,” a synthetically produced but chemically identical form of exendin-4, as a medication for people with type 2 diabetes. Because “exenatide” cannot be absorbed in pill form, a pharmaceutical company developed an easy-to-use injection “pen” (not unlike the one later approved for administering emergency glucagon) that patients could use to give themselves the correct dose of the drug. In industry-supported randomized controlled clinical trials, this approach proved highly beneficial in participants with type 2 diabetes whose blood glucose was inadequately controlled. At the conclusion of the studies, participants who had received exenatide were found to have maintained significantly healthier blood glucose levels than those who had received a placebo; and those receiving a higher dose of exenatide had achieved better blood glucose control than those who received a lower dose. Because it does not directly increase insulin levels, but rather signals β cells to be ready to produce a robust insulin response if it is needed, those taking it did not tend to experience hypoglycemia. The drug therefore also proved to be a relatively safe method for improving blood glucose control. As a result, the FDA approved exenatide in 2005 as a supplementary treatment for type 2 diabetes in patients whose blood glucose is not otherwise well controlled.
New Benefits and a New Indication for the New Class of Medicines
Subsequent FDA approvals went to even longer-lived forms of GLP-1, like liraglutide, which lasts 24 hours, and semaglutide, which lasts an entire week. Together, these medications constitute a distinct and entirely new class of medications for type 2 diabetes called “GLP-1 receptor agonists.” Because the FDA wanted to be sure that these drugs help people to control their blood glucose levels without incurring risks that might worsen cardiovascular disease, the diabetes complication most likely to result in death, the agency ordered post-approval research of long-term outcomes in people taking them. As a result, we now know that the medicines in this class significantly reduce the risk for heart attacks and other cardiovascular complications that are the leading killers of people with diabetes.
Another exciting finding from randomized clinical trials of these therapeutics is that people taking these medications typically lose a significant amount of weight. This “side effect,” of course, is quite valuable, because type 2 diabetes is associated with overweight and obesity. These findings spurred interest in testing GLP-1 receptor agonists for another purpose: treating obesity. Indeed, clinical trials have indicated that they are remarkably effective as weight-loss medications. Depending on the dose, for example, weekly semaglutide treatment led to an average weight loss of about 16 percent within 1 year in people with obesity who did not have diabetes, while being relatively safe for many people and fairly well tolerated.
Thus, basic research on the hormonal control of blood glucose levels, studies in animals as different as deep-sea fish and desert-dwelling lizards, and creative innovations in drug stabilization and delivery have helped lead to the development and FDA approval of two new classes of medication— glucagon and GLP-1 receptor agonists. These medications have very different effects, but together they are improving care and health for millions of people: reducing the risk of hypoglycemia in people with type 1 diabetes, improving blood glucose control in people with type 2 diabetes, and yielding weight loss for people with obesity.